Introduction
SPAD (Single Photon Avalanche Diode) image sensors are highly sensitive photodetectors capable of detecting single photons.
With a level of sensitivity and time resolution unattainable by conventional CMOS or CCD image sensors, SPAD technology is gaining attention as the next generation of imaging technology. This article provides an in-depth explanation, covering the fundamental principles of SPAD image sensors, their technical features, applications, current challenges, and future prospects.
1. Basic Principles of SPAD Image Sensors
1.1 What is the Avalanche Multiplication Effect?
SPAD detects photons by utilizing the avalanche multiplication effect in semiconductors.
- Avalanche Multiplication Effect: In a semiconductor under a high electric field, a photon generates an electron-hole pair. These carriers are accelerated, causing them to generate additional carriers through impact ionization, resulting in a chain reaction of multiplication.
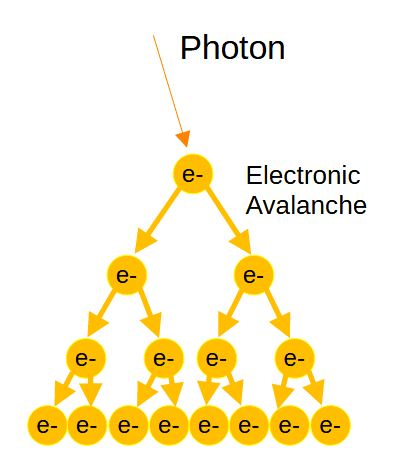
1.2 Operating Principle
The operation of SPAD can be explained through the following steps:
- Setting the Overvoltage State: SPAD operates in an overvoltage state, where the applied voltage exceeds the semiconductor’s breakdown voltage. This condition facilitates avalanche multiplication, even with a single photon.
- Photon Absorption and Carrier Generation: An incoming photon generates an electron-hole pair within the semiconductor.
- Initiation of Avalanche Multiplication: The generated carriers are accelerated by the strong electric field, triggering avalanche multiplication as they create additional carriers.
- Signal Detection: The amplified current is extracted as a pulse signal by the detection circuit.
- Quenching (Stopping the Avalanche): To prevent the avalanche phenomenon from persisting, the quenching circuit temporarily reduces the voltage, stopping the avalanche process.
1.3 Quenching Mechanism
- Passive Quenching: This method uses a resistor to naturally decrease the current and stop the avalanche. While the circuit is simple, it results in slower response times.
- Active Quenching: This approach employs a switching circuit to forcibly lower the voltage, quickly halting the avalanche. It is suitable for applications requiring high-speed response.
Technical Features of SPAD Image Sensors
2.1 Single-Photon Sensitivity
SPAD has extremely high sensitivity as it can detect single photons. This feature is particularly useful for applications involving low light levels.
2.2 High Temporal Resolution
- Picosecond-Level Temporal Resolution: SPAD responds extremely quickly, allowing it to record the exact time a photon is detected with high precision.
- Time-Correlated Single Photon Counting (TCSPC): Using this technique, SPAD can perform high-precision temporal analysis by statistically measuring photon arrival times.
2.3 Pixel Array Configuration
- Individual SPAD Elements in Each Pixel: A SPAD image sensor consists of a two-dimensional array of numerous SPAD elements.
- Integration of Peripheral Circuits: Quenching circuits, counters, and timing circuits are integrated into each pixel.
2.4 Noise Characteristics
- Dark Count Rate (DCR): Even in the absence of light, false detections can occur due to thermal excitation. Material selection and temperature control are crucial for reducing this effect.
- Afterpulsing: This phenomenon occurs when trapped carriers from a previous avalanche trigger another avalanche, causing noise. Detection is controlled by introducing a temporal window.
2.5 Crosstalk Issues
- Optical Crosstalk: This phenomenon occurs when photons generated by an avalanche in one pixel travel to adjacent pixels, causing false detections.
- Electrical Crosstalk: Sudden current changes induce noise in the circuit, affecting the operation of other pixels.
- Countermeasures:
- Optical: Incorporate light-blocking walls between pixels.
- Electrical: Optimize the circuit design
3.Applications of SPAD Image Sensors
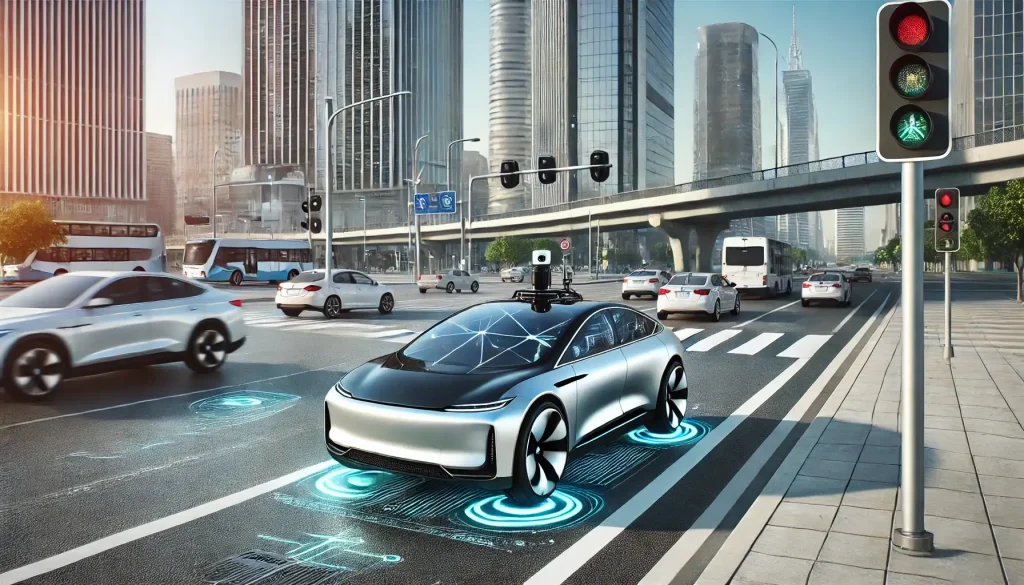
3.1 LiDAR (Light Detection and Ranging)
- Distance Measurement: By emitting light pulses toward a target and measuring the time it takes for the reflected light to return, distances can be calculated.
- High-Resolution 3D Mapping: The fast response of SPAD enables capturing the surrounding environment in three dimensions with high spatial resolution.
- Applications in Autonomous Driving: Accurate real-time distance information contributes to the development of safe autonomous driving systems.
3.2 Biomedical Imaging
- Fluorescence Lifetime Imaging (FLIM): Measures the emission lifetime of fluorescent substances to analyze the state and environment of biomolecules.
- Single-Molecule Detection: Detects extremely weak fluorescence signals to observe the dynamics of DNA and proteins.
- Advantages: Enables non-invasive, highly sensitive measurements, reducing the burden on biological samples.
3.3 Quantum Communication and Quantum Cryptography
- Quantum Key Distribution (QKD): Enables secure information transmission at the single-photon level, ensuring advanced security.
- Role of SPAD: As a highly sensitive single-photon detector, SPAD is essential for the accurate detection of quantum states.
3.4 Astronomy and Space Exploration
- High-Sensitivity Observations: Detects faint light from distant stars and galaxies.
- Time-Resolved Observations: Supports the study of rapidly changing celestial phenomena, such as pulsar observations.
Technical Challenges and Solutions
4.1 Reducing Dark Count Noise
- Cryogenic Operation: Lowering the operating temperature suppresses thermal excitation, reducing the dark count rate.
- Material and Process Optimization: Using high-quality semiconductor materials and refined manufacturing processes minimizes defects.
4.2 Higher Integration and Improved Fill Factor
- Fill Factor: The ratio of the pixel area effectively used for light detection.
- Countermeasures:
- Use of Microlenses: Focuses incoming light onto the effective detection area.
- 3D Stacking Technology: Vertically arranges circuits and detection elements to improve area efficiency.
4.3 Power Consumption Issues
- Power-Saving Design: Reduces power consumption through circuit optimization and low-voltage operation.
- Power Management: Implements control mechanisms to activate circuits only when needed.
4.4 Suppression of Crosstalk and Afterpulsing
- Light-Blocking Wall Design: Physically blocks optical interference between pixels.
- Circuit Timing Optimization: Operates at timings that minimize the likelihood of afterpulsing.
5.Latest Research Trends and Future Prospects
5.1 Higher Resolution
- Pixel Size Reduction: Advances in microfabrication technology allow more pixels to be integrated into a single sensor.
- Challenges: Increased crosstalk and noise between pixels necessitate highly precise design techniques.
5.2 Multispectral Imaging
- Simultaneous Detection of Multiple Wavelength Bands: Combines SPADs sensitive to different wavelengths to analyze color information and material properties.
- Applications: Remote sensing, medical diagnostics, environmental monitoring, and more.
5.3 Integration with AI
- On-Chip Signal Processing: Performs data analysis directly within the sensor, enabling real-time extraction of useful information.
- Use of Machine Learning: Applies AI algorithms for noise reduction and pattern recognition.
6.Conclusion
SPAD image sensors, with their exceptional sensitivity and temporal resolution, are driving innovation across a wide range of fields. While technical challenges remain, advancements in research and development are steadily addressing these issues. Going forward, SPAD technology is expected to play a pivotal role in measurement, communication, and image processing, significantly impacting our lives and industries.
コメント